Abstract
A flowback procedure is presented that uses a wellbore model coupled with thermal and fluid modeling to calculate the bottomhole pressure (BHP) and maximize the safe initial production/drawdown without over-stressing and damaging the fracture system. After obtaining the BHP, it is then possible to determine the fractured reservoir volume, fracture-dominated volume, and matrix contribution. The paper presents a case history validating the model calculations in an unconventional well. Two methods of decline analysis are presented, ‘Conventional’ and ‘Thermodynamic Transient Analysis’ (TTA), and together, these methods provide insights into the fracture system and overall reservoir volumes by recognizing and applying the appropriate equation for the proper reservoir flow regime. The early flowback period can be used to determine the frac volume, while later periods will begin showing the increasing amount of matrix contribution. The wellbore flow model and decline analysis calculations are verified by a case history presented in the paper, where this flowback process was utilized on a hydraulically fractured East Texas well. The wellhead pressures (WHPs) in this case study highlight the need to accurately calculate BHPs based on the WHP and rates, due to significant differences between the trends and decline rates of the respective pressures.
Introduction
An operator’s key objective during a frac flowback is often to bring the well online with a high initial production rate to demonstrate high productivity capabilities to investors. Without the appropriate engineering safeguards, this objective is susceptible to overstressing the proppant and damaging the long-term hydrocarbon recovery of the well. This paper presents an engineered frac flowback workflow that is designed to maximize the safe initial production while avoiding over-stressing the fracture system. This procedure requires bottomhole pressures (BHPs) to be accurately calculated, allowing monitoring of fractured reservoir volumes, fracture-dominated volumes, and matrix contribution. A case study of an East Texas well is presented to demonstrate this workflow.
Methods
The first part of the workflow is to calculate accurate BHPs using a direct solution to the Mechanical Energy Balance Equation, utilising a wellbore model coupled with a phase and thermal-transient three-phase flow model (Fair et al. 2014). Calculated BHPs can then be used to perform two methods of decline analysis, ‘Conventional’ and ‘Thermodynamic Transient Analysis’ (TTA), to monitor reservoir volumes by applying the appropriate equation for the proper reservoir flow regime.
Conventional decline analysis can be used to calculate the connected volume via the following equation (Fair et al. 2014):
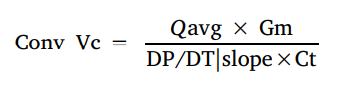
Conv Vc = Connected compressibility volume (bbl)
Qavg = Average flow rate over selected interval (bbl/day)
Gm = Geometric multiplier dependent on flow regime
DP/DT-slope = Rate of pressure decay (psi/day)
Ct = Total compressibility (1/psi)
Conv Vc characterises the fluid volume that is hydraulically connected back to the wellbore in an expansion drive system. Not all the hydraulically connected volume is actively moving to the well. To determine the mobile volumes, TTA decline analysis must be performed by applying the following equations (Fair et al. 2014):
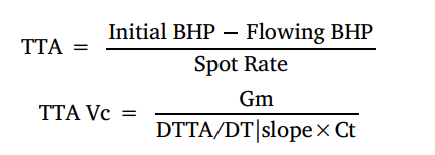
TTA Vc = Mobile compressibility volume (bbl)
Gm = Geometric multiplier dependent on flow regime
DTTA/DT-slope = Rate of TTA decay (psi/(bbl/day)/day)
Ct = Total compressibility (1/psi)
The volumes calculated from conventional and TTA decline analysis can be monitored throughout the flow back to assess the completion and ensure the proppant is not being over-stressed. These volumes will generally increase over the course of the flow back as the reservoir transitions into a different flow regime and more fluid is hydraulically connected and mobilized toward the well. A decrease in these volumes indicates that too much stress is being placed on the proppant, leading to compaction and/or crushing and that the choke should be reduced to lessen the stress on the fracture system.
When initially bringing these wells online, they are dominated by water production. Decline analysis is performed with the water rates to calculate volumes until the well begins transitioning to produce hydrocarbons. In the first few hours, the reservoir will be in a frac flow regime, only seeing energy from the high-permeability fractures and characterized by a steep decline in BHP. Shortly after the frac flow regime, the reservoir will transition to linear horizontal flow, with a shallower decline in BHP representing a gain in volume as the well sees more energy from the near-fracture region. Finally, the well transitions to a linear matrix flow as it starts to pull in more fluids from the unstimulated rock matrix. Again, as more energy from the surrounding rock is seen by the well, volumes grow, and the rate of decline in BHP reduces.
Over the course of the flow back, decline analysis volumes will typically grow as the reservoir progresses through these flow regimes, and the well sees more energy and fluid contribution from the system. If these volumes start to decrease, this is an indication that too much stress is being placed on the fracture system, and the choke should be reduced to avoid further damage to long-term production.
Results
This flowback process was performed on a hydraulically fractured East Texas well. The wellhead pressure (WHP) in this case study highlights the need to accurately calculate BHP, as the trends and decline rates observed in the WHP differ significantly from those observed in the BHP, shown in Fig. 1, due to changes in fluid density throughout the wellbore. As the flowback continues, the well gradually produces more and more gas. More gas in the wellbore reduces the hydrostatic head and reduces the decline rate of WHP compared to BHP, actually raising WHP during several gas surges and stage clean-up events. Attempts to calculate and monitor volume changes based on the WHP would not be valid due to the wellbore density changes masking the actual reservoir response.
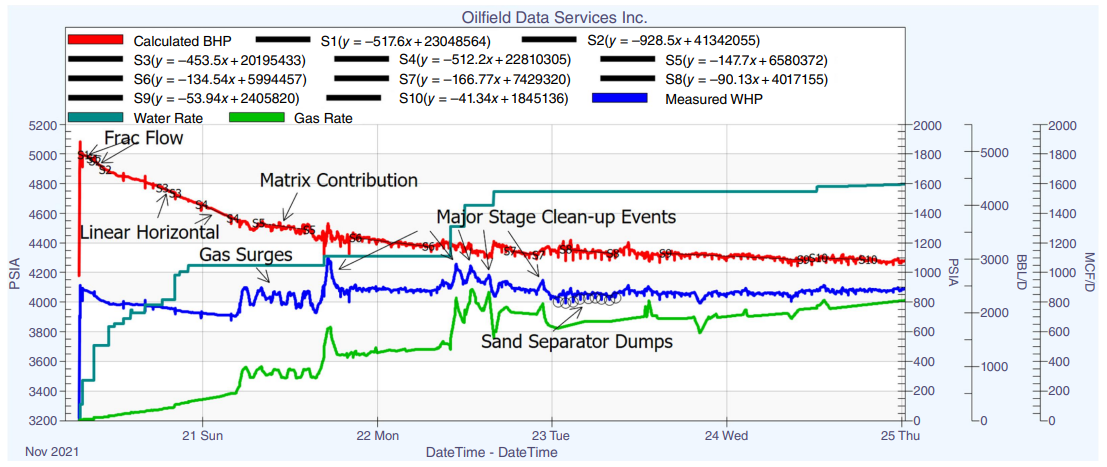
Figure 1
Data from a flowback of a hydraulically fractured East Texas well. Calculated BHP is on the left axis, Measured WHP on right axis 1, Water Rate on right axis 2, and Gas Rate on right axis 3.
This well was brought online at a 12/64-inch choke and flowed at that setting for 4 hours to establish a baseline frac volume, then the choke was then increased to 16/64-inch to generate a new transient and enable another look at the frac volume. Table 1 shows a slight reduction in the calculated volumes from slope 3 to 4 due to choke increases during this period. The team decided to hold the choke constant and to discuss if choking back was necessary with the day hands during shift change. Just before shift change, the reservoir entered the matrix contribution flow regime, BHP declined at a lower rate, and more energy and volume were seen by the well. It was then determined to proceed with choke increases as long as the observed volumes continued to grow.
Water rate (Qw) was used to calculate connected volumes as it was the dominant phase during the early flowback. Rates were converted to a total reservoir rate (Qtot) to calculate the TTA function and ensure mobile volumes incorporated the effect of both water and gas rates.
While reservoir volumes can be calculated and monitored using BHP and conventional decline, it can be harder to intuitively interpret the volume. A steeper decline in BHP does not necessarily result in a reduction in volume, as it could be counteracted by an increase in rates. Observing the TTA allows for a much more intuitive view of how volumes are changing over the course of the flowback as a steeper slope more directly translates to less volume.
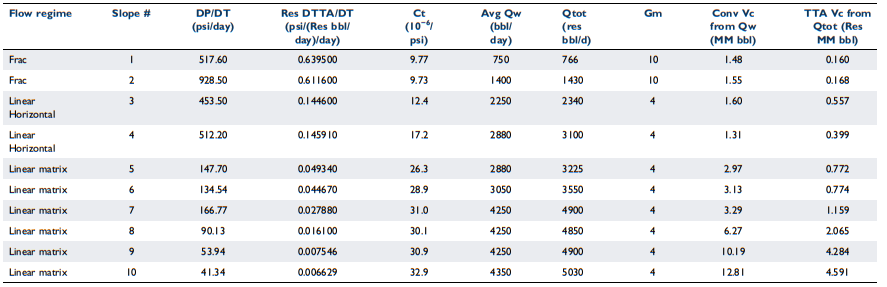
Table 1
Calculated connected and mobile volumes during the flowback of a hydraulically fractured East Texas well.
Viewing a plot of the TTA, shown in Fig. 2, shows a clear picture of the different flow regimes and changes in volume during the flowback. Grouping similar TTA slopes also helps identify the flow regime for the reservoir. Similar slopes on T1 and T2 show the reservoir in its frac flow regime. A volume gain in slope T3 indicates the reservoir has transitioned to linear horizontal flow. Further slope decreases to T5 and beyond show that the reservoir is gaining more and more matrix contribution with increasing volumes. The lack of sharp increases in the TTA slope shows that this flowback was conducted without over-stressing the proppant and fracture system, ensuring that long-term production is not adversely affected by the initial flowback.
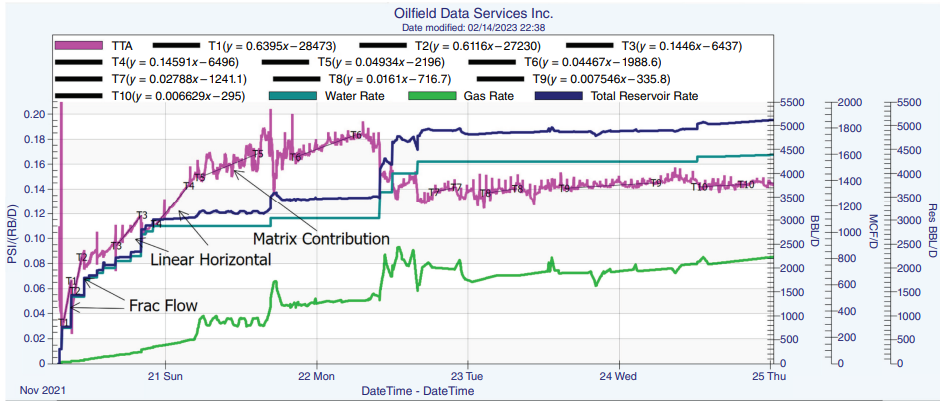
Figure 2
Calculated TTA from flowback of a hydraulically fractured East Texas well.
Summary and Conclusion
Frac flowbacks play a pivotal role in the production of hydraulically fractured, unconventional wells. While providing a chance to boast high initial production and attract investors, there is also the risk of flowing the well too hard, damaging the fracture system, and reducing ultimate recoverable volumes. The decline analysis methods presented are focused on providing the size of the frac-dominated volume and the matrix volume as early as possible in the well flowback test. This procedure also allows for continued evaluation of the reservoir volumes as production continues. Managing the frac flowback via conventional and TTA decline analysis, calculated from accurate BHPs, effectively safeguards against the risk of over-stressing the proppant and allows operators to safely maximize initial production while protecting long-term recovery.
Reference
Fair C, Flores R, Hakim B, Nande S (2014) Using the Results from Automated Petroleum Engineering Calculations to Accelerate Decision Workflows. Paper presented at the SPE Asia Pacific Oil & Gas Conference and Exhibition, October 2014, Adelaide, Australia. (Society of Petroleum Engineers) doi:10.2118/171512-ms